It’s about as audacious an idea as you can find in the world of infectious disease: a broad-spectrum antiviral — a “penicillin for viruses.”
The stakes are incredibly high. The yearly tide of seasonal flu causes three to five million severe cases annually, killing hundreds of thousands of people. The “break-bone fever” of dengue virus threatens roughly half the world’s population. 350 million people today are living with hepatitis B or C, leading to millions of cases of liver disease and cancer. The HIV and COVID-19 pandemics have already killed tens of millions, and viruses from Ebola to bird flu are constantly threatening future epidemics, too.
All told, viral infections will account for tens of millions of deaths and trillions in economic losses over the coming decades. A drug that could treat all of them — or even a broad class of them — would be huge.
However, making any antiviral is hard enough, biochemist, former rocket scientist and software engineer, and serial entrepreneur Rick Kiessig explains.
Viral infections will account for tens of millions of deaths and trillions in economic losses over the coming decades. A drug that could treat all of them — or even a broad class of them — would be huge.
Viruses have sophisticated defenses, developed over eons of evolution. When they are inside cells, it’s generally too late. When they’re outside of cells, these tiny invaders are difficult to spot. And one favorite drug technique — attacking the biochemical pathways of organisms — does not work on viruses; they aren’t even alive.
“There’s something like 219-ish known diseases in humans that are caused by viruses,” Kiessig tells Freethink — and out of these hundreds, there are only a handful with antiviral treatments.
“So yeah, it’s a hard problem.”
The New Zealand-based startup Kimer Med, where Kiessig is the co-founder, chief science officer, and CEO, is taking on the ambitious mission of solving that problem. Inspired by work published in 2011, they are trying to develop a broad-spectrum antiviral that uses a target in human cells to hopefully attack a wide variety of viruses.
The technology behind it has already failed to gain traction once, but Kimer is betting that this time — in a post-pandemic world — will be different.
An inspiration: The previous approach, called DRACO, and Kimer’s new candidate, called VTose (pronounced “vee-tohs”), both hinge on something called double-stranded RNA. But while their targets are the same, VTose uses different proteins to form its virus-fighting “toolkit,” as Kiessig puts it.
Double-stranded RNA, or dsRNA, is a signature of virus replication in cells, a signal that most viral infections create.
The new design, VTose, recognizes the dsRNA and triggers a process called apoptosis, programmed death, in the infected cell — hopefully stopping the infection in its tracks.
While ambitious, a broad-spectrum antiviral based on this technique is not an impossible goal.
The potential broad-spectrum antiviral takes aim at a signature of viral replication inside host cells: long strings of double-stranded RNA.
Apoptosis is “a well understood process,” Mark Hickman, a senior scientist with a component of the US Department of Defense’s Chemical and Biological Defense Program, tells Freethink. Hickman and colleagues helped develop relatively broad-spectrum antivirals against filoviruses, like Ebola and Marbrug, which culminated in the drug remdesivir (which was later repurposed to fight COVID-19).
Taking aim at dsRNA to make a broad-spectrum antiviral “sounds plausible,” Hickman says, with the caveat that more data, including clinical data, is needed.
The dsRNA mechanism has already shown some promise. In 2011, Todd Rider — a polymath with multiple degrees from MIT, then affiliated with MIT’s Lincoln Laboratory — and colleagues published the initial DRACO paper as proof-of-concept.
In those initial studies, the Rider Institute explains, DRACO was found to be effective against 18 different viruses in 13 different kinds of mammalian cells, including type 2 dengue virus, H1N1 influenza, and multiple rhinoviruses and coronaviruses, which cause the common cold. It was also found to be nontoxic in mice and protected or rescued them from a number of virus challenges, including H1N1 influenza.
It was an exciting find that was met with excitement in the popular press. Bloomberg declared that “Todd Rider Has a Kill Switch for Viruses,” and The Verge called DRACO a “doomsday device” against viruses. While certainly not a guaranteed slam dunk, it looked worth further investigation; unfortunately, that never came, as financing dried up and DRACO ended up in the research “valley of death,” between basic science and clinical testing.
In August 2021, a year after its founding, Kimer Med licensed the final DRACO-related patent from MIT and started building their own broad-spectrum antiviral.
The new contender: Kimer’s drug is unlike most antivirals, which use small molecules. Instead, VTose is a large, complex molecule, built from pieces of proteins that are present in most of our cells.
Large and small compounds have different advantages and drawbacks. While they can cost more to develop, Kiessig says, small molecule antivirals are cheaper and easier to make than large molecules. But their antiviral abilities can cut both ways. Small molecule compounds have a history of being “relatively toxic” to healthy cells, Kiessig says — hence the expensive initial development.
“It requires a lot of work, a lot of different trials — hundreds of different compounds, potentially — to try to find a nontoxic small molecule that both interferes with the pathway you want it to interfere with,” Kiessig says, “and doesn’t kill the patient in the process.”
Large molecule compounds have a better safety reputation, but are more difficult and expensive to manufacture.
The new design, VTose, recognizes dsRNA and triggers a process called apoptosis, programmed death, in the infected cell — hopefully stopping the infection in its tracks.
Targeting the host: Broad-spectrum antivirals fall into two different categories: antivirals that target the viruses directly, and antivirals that target the cells viruses infect and use for replication. Viruses are incredibly diverse, so finding a drug that directly works against a wide range of them would be a very difficult task.
Instead, VTose falls into the latter category, called “host-directed antivirals” (HDAs). An HDA has a few benefits that make it a potentially ideal choice for a broad-spectrum antiviral.
Because it is host-directed, it should be more difficult for viruses to evolve their way around it — the bane of all antiviral treatments, from COVID-19 to HIV.
“We believe [how VTose works] is much more resistant to adaptation by the virus,” Kiessig says. “Because what we’re doing is actually attacking something that’s common across pretty much all viruses … we’re attacking a basic aspect of viral replication.”
A broad-spectrum HDA has another possible benefit: it could be developed before a new virus emerges.
“A pre-existing repertoire of first-line, broad-acting HDAs that can be readily deployed may be beneficial in slowing the initial viral spread or in suppressing outbreaks,” Boston University researcher Vipul C. Chitalia and drug discoverer Ali H. Munawar wrote in the Journal of Translational Medicine. “Later, HDAs can be complemented with [direct-acting antivirals] and vaccines since their development hinges on the knowledge of specific viral proteins.”
But to do that, a broad-spectrum antiviral needs a target that is common across many kinds of viruses — across viruses that infect different kinds of cells, in different ways — and is unlikely for the virus to mutate around. Enter double-stranded RNA.
“It is exactly the same sort of thing that the innate immune system tries to do.”
Rick Kiessig
How VTose works: VTose works by taking aim at dsRNA.
According to researchers Y. Grace Chen and Sun Hur, at Yale and Harvard respectively, dsRNA is present in the majority of viral infections. It can come from the viral genome itself, or it can be created inside of cells during viral replication.
“Hence, nearly all organisms have the capability of recognizing dsRNA and mounting a response, the primary aim of which is to mitigate the potential infection,” they wrote.
Unfortunately, viruses are good at what they do and can flummox the efforts of the innate immune system, the first line of defense in cells that recognizes dsRNA.
VTose works by recognizing the long strings of dsRNA that occur when a virus tries to replicate inside a cell. The large molecule then triggers the infected cell’s natural suicide sequence — apoptosis — to protect the rest of the body.
“It is exactly the same sort of thing that the innate immune system tries to do,” Kiessig says.
The first part of VTose’s process uses the same protein that cells naturally use to detect viruses, a piece of an enzyme called PKR. When it detects an infection, VTose then uses part of a protein called Apaf-1, which kicks off a biochemical chain reaction that results in apoptosis, killing the cell before the virus can use it to replicate and spread the infection.
“You put those two things [together], and the idea is that now we have a protein that detects dsRNA … and it kills the cell,” Kiessig says.
Thus far, VTose has shown success against dengue and “a handful of other viruses” in cells — a first step on a long, treacherous path to clinical trials and, if it works there, hospitals.
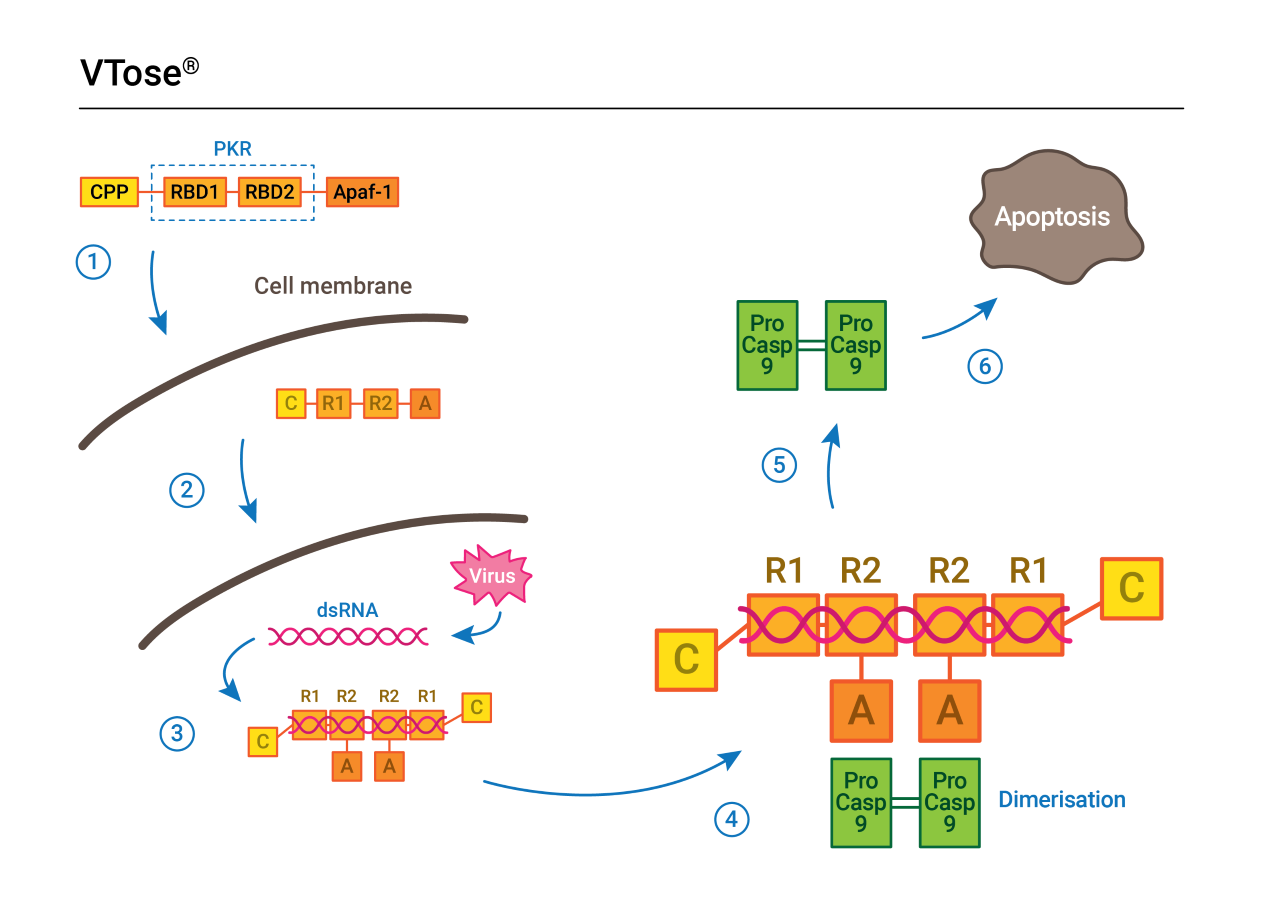
The challenges: Multiple hurdles stand in the way of that, not the least of which is whether this drug even works at stopping viruses in humans at all — rather than Petri dishes or mice.
“The majority of phase 1 drugs and therapeutics die in phase 1,” Hickman, the DoD senior scientist, says.
There’s good animal models for infectious disease — mice and monkeys get infected by viruses in a very similar way to humans — but many drugs die because they are simply, and unexpectedly, too toxic. Proving that a broad-spectrum antiviral is not toxic at the doses it needs to work will be one hurdle to cross.
While large molecules tend to be less toxic, they are more difficult and expensive to manufacture, and they face another barrier: the regulatory pathway for small molecule antivirals is better established. That means it’s clearer what you need to do to convince the FDA the drug is safe and effective — what experiments and data you need to collect, how the trials need to be designed and run, and so on.
“They understand it really well, and how it’s meant to work from end to end,” Kiessig says. There are approved large molecule drugs, but the paths are not as well trod — and that’s a problem for a small startup.
Of course, everything hinges on a question that can only be answered by reaching the stage DRACO never did: does it work in humans?
It’s a radical, ambitious idea — the kind that naturally draws healthy, warranted skepticism. But if it does, VTose could be a powerful new weapon for public health. Kiessig is excited by the prospect.
“The opportunity here is tremendous,” Kiessig says. “If we’re successful, we have an opportunity to make a change that’s as significant as broad-spectrum antibiotics were for the world.”
We’d love to hear from you! If you have a comment about this article or if you have a tip for a future Freethink story, please email us at tips@freethink.com.